Robotic engineers have favored the straightforwardness of lizard-like tails, however, tails resembling those of mammals might be both lighter and more efficient for maneuvering a robot’s body in three-dimensional space
During their investigation into the optimal design of robots that utilize tails for body reorientation in midair, a group of scientists from the University of Michigan and University of California San Diego uncovered that mammals had already mastered the art of achieving more with fewer resources.
The researchers assert that their simulation technique will guide the creation of robots equipped with lighter yet more efficient tails—and reveal how animals apply physical principles to navigate.
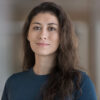
“Nature essentially developed two categories of vertebrate tails. The robust, muscular tails of lizards enable body reorientation in a singular rotational plane and have influenced numerous rigid-tailed robots,” stated Talia Moore, an assistant professor of robotics at U-M and the lead author of the research published in the Journal of the Royal Society Interface.
“Initially, the lightweight, tendon-driven tails of mammals such as cats appeared to lack effectiveness in body rotation. However, we discovered that mammal tails have the ability to create intricate, three-dimensional curves, and many mammals seem to be proficient at inducing body rotations with their tails.”
Over a decade ago, Moore attempted to determine if jerboas, long-tailed desert rodents that move by hopping on two hind legs, utilized their tails for this purpose. Nevertheless, the equation devised to describe tail motion dismissed the curving and whipping nature of mammalian tails—and it claimed that no jerboa could possibly swing its tail swiftly enough to rotate its body. She set the inquiry aside.
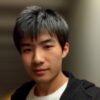
Then, Xun Fu, a graduate student in Moore’s team at that time, aimed to create a robot with a tail. They revisited the issue with detailed computer simulations focused on understanding how the joints of the tail influence the capacity to rotate a body in space. The researchers claim their study is the first to evaluate the efficiency of various complex appendages for twisting and turning within three-dimensional environments through simulations.
Specifically, they sought to determine if augmenting the number of bone segments and altering their lengths would improve a tail’s capability for body rotation. The team devised challenges: using only tail motion, a box-like body needed to reposition itself, flipping and rotating in a zero-gravity environment.
Each tail was assessed based on how well it enabled the body to achieve each target position. To ensure equitable conditions for all tails, the researchers restricted the total effort allowable for repositioning—in tails with multiple segments, the cumulative effort across all joints matched the maximum effort produced at the single joint controlling the one-segment tail.
“We were unsure what the results would reveal when exploring the impact of different tail joint configurations, particularly when allowing for the length of individual bones to be varied as part of the optimization process,” said Fu, the primary author of the research and now a doctoral graduate in robotics.
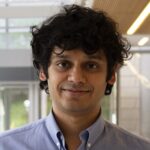
With assistance from Ram Vasudevan, a professor of robotics at U-M, and robotics doctoral student Bohao Zhang, the team utilized the model to uncover the optimal tail structure for inducing body rotations: It featured the greatest number of segments, commencing with a short bone, rapidly extending to the longest bone, and then gradually reducing in length towards the tail’s tip.
Moore and Fu then engaged collaborators at UCSD, who were examining museum specimens of mammal tail bones. Within the UCSD findings, nearly all mammals that rely on mid-air reorientation exhibited a similar pattern of bone lengths.
“The skeletal structures of mammal tails are vastly different from one another, and now we can affirm that this particular type of tail evolves to facilitate inertial maneuvering. We’re eager to explore how other tail types function,” stated Ceri Weber, a postdoctoral researcher collaborating with Kimberly Cooper, a UCSD professor specializing in cell and developmental biology.
Moore proposes that simulations similar to theirs could be expanded to assess the efficiency of moving limbs, wings or legs in intricate, three-dimensional shapes for twisting and turning in midair, maintaining equilibrium, and beyond. The findings could provide insights into the biomechanics of humans and animals, while also directing the design of robots.
“The surge and decline in length distribution pattern observed in the optimized tails, reminiscent of certain mammal tails noted by biologists, was both astonishing and thought-provoking,” Fu remarked. “In some respects, this underscores just how much more we still need to learn about these creatures to truly connect the realms of biophysics and robotic capabilities.”
The research was conducted with support from various discretionary funding awards: the Oak Ridge Associated Universities Ralph E. Powe Junior Faculty Enhancement Award (Moore), the Ruth L. Kirschstein National Research Service Award Individual Postdoctoral Fellowship (Weber), the Wu Tsai Human Performance Alliance and the Joe and Clara Tsai Foundation (Cooper).