Science & Tech
Suggesting a resolution to a chicken-or-egg dilemma in evolution
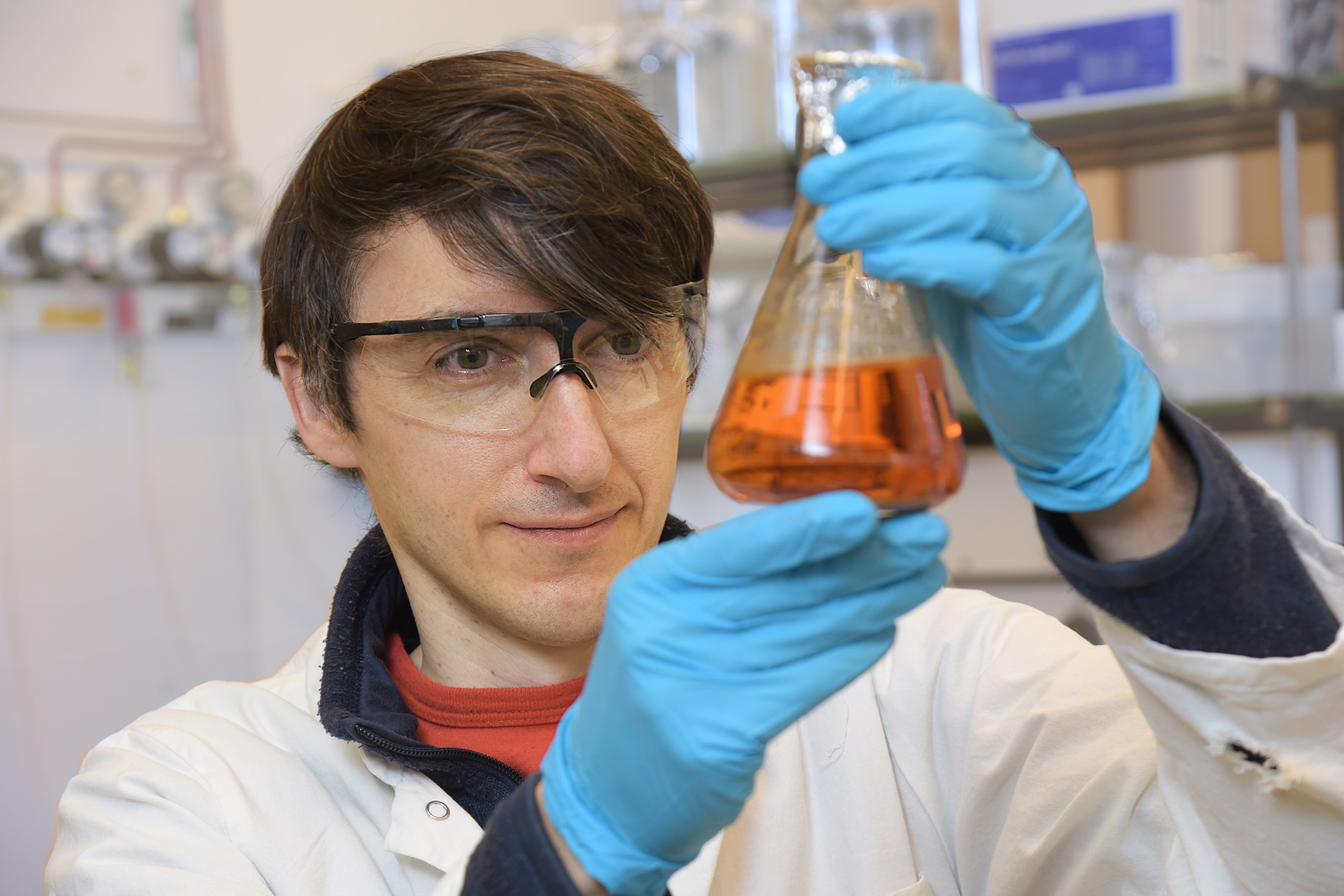
Felix Elling, a past postdoctoral researcher at Harvard and the principal author of the study.
Image courtesy of Felix Elling
A serendipitous discovery might assist researchers in determining which came first: the capacity to generate oxygen through photosynthesis or to utilize it via aerobic metabolism
For biochemists, it’s the classic query of which came first: oxygen generation through photosynthesis or oxygen utilization through aerobic metabolism?
During photosynthesis, algae and plants absorb sunlight to convert carbon dioxide and water into energy for growth, releasing oxygen as a byproduct. Conversely, animals consume oxygen to transform the fuel ingested into energy and produce carbon dioxide, a process known as aerobic metabolism.
So, which occurred first? A recent study published in the Proceedings of the National Academy of Sciences outlines an unintentional find by a global group of researchers of a potentially missing-link molecule that might provide clarity on this evolutionary query.
“From the beginning, we suspected this could be tied to the evolution of photosynthesis and the capacity to utilize oxygen,” stated Felix Elling, a former postdoctoral researcher in the Department of Earth and Planetary Sciences and main author of the paper.
Elling, who was engaged in research at Professor Ann Pearson’s Lab for Molecular Biogeochemistry and Organic Geochemistry, was investigating particular molecules unrelated to the evolution of aerobic metabolism when he stumbled upon something unexpected: a minor alteration in a molecule within a nitrogen-utilizing bacterium, Nitrospirota, that resembled a molecule needed by plants for photosynthesis rather than by a bacterium.
“We were screening bacteria for a completely different objective,” remarked Elling, who now serves on the faculty at the University of Kiel in Germany.
The discovery made by the researchers was methyl-plastoquinone, a variant of a molecule class known as quinones. Present in all organisms, quinones were previously thought to exist in two fundamental types: aerobic quinones that necessitate oxygen and anaerobic types that do not.
Aerobic quinones can be further categorized into two types — one utilized by plants for photosynthesis and the other by bacteria and animals for oxygen consumption.
“Essentially, all forms of life depend on quinones for their metabolic processes,” described Elling. Discovering a quinone that is “similar to those used by plants for photosynthesis” within an oxygen-breathing bacterium was quite extraordinary. Researchers soon realized that methyl-plastoquinone represented a third type and could potentially be a missing link connecting the two.
“This molecule serves as a time capsule. A living fossil of a molecule that has persisted for over 2 billion years.”
Felix Elling
The study illuminates what is referred to as the Great Oxidation Event. This era — approximately 2.3 to 2.4 billion years ago — signifies when cyanobacteria (a specific type of algae) began producing substantial amounts of oxygen through photosynthesis, enabling aerobic metabolism.
Although this advancement suggests that photosynthesis preceded oxygen utilization, the identification of methyl-plastoquinone bolsters an alternative theory. In simple terms, some bacteria were capable of using oxygen even before cyanobacteria began its production.
In other terms, “the chicken and the egg were present simultaneously,” stated Elling.
Pearson, the PVK Professor of Arts and Sciences and the Murray and Martha Ross Professor of Environmental Sciences, in whose lab Elling’s research was initiated, emphasized the significance of having a biochemical mechanism for processing oxygen at the time its generation by photosynthesis started.
“The reactions involving oxygen can be very harmful and potentially lethal to cells lacking mechanisms to manage the metabolic byproducts,” she explained. While we often take them for granted, “the biochemical systems that we utilize in our cells to sustain our aerobic metabolic existence are quite intricate.”
In brief, “this is how we came to respire,” Pearson declared. “And once we are able to breathe oxygen safely, it opens the door for the proliferation of the diverse life forms we observe today.”
Traces of the evolution of quinone structures can be detected in our own bodies, highlighting the fundamental differences between quinones present in human mitochondria compared to those found in plants.
“We believe that our finding represents the primary or ancestral version of this molecule that subsequently adapted into two forms—one with specific roles in algae and plants, and the alternative form in mitochondria present in our bodies today,” Elling explained.
“This molecule acts as a time capsule,” affirmed Elling. “A living fossil of a molecule that has survived for over 2 billion years.”
This study received partial funding from the U.S. National Science Foundation.