“`html
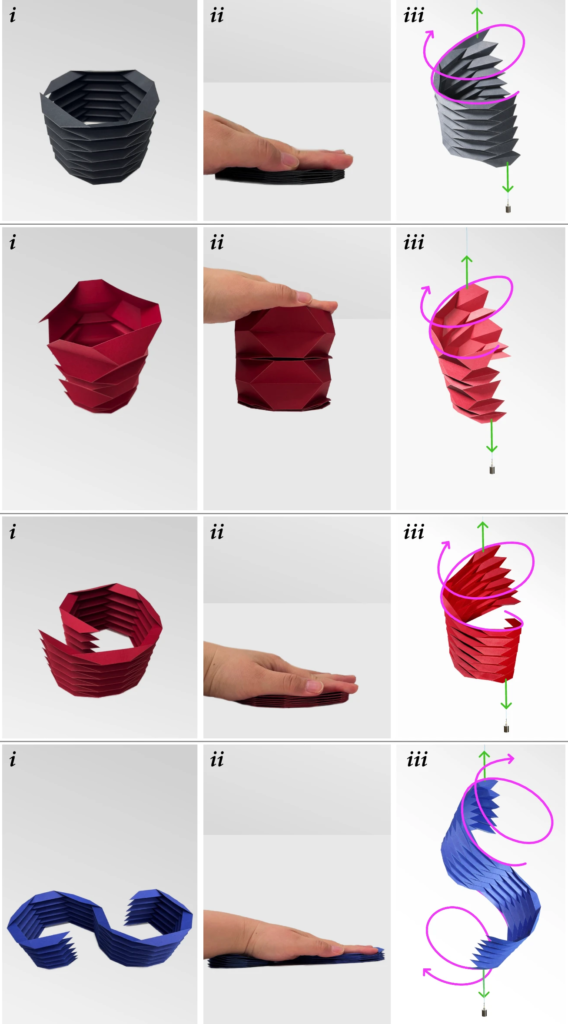
Origami—the craft of paper folding that began in Japan centuries back—may unveil a new realm in progressive materials, propelled by studies led at the University of Michigan.
As an art form, origami employs basic folding methods to craft elaborate designs. Currently, researchers are examining this technique as a basis for future materials that can be expected to deform and “fold” under appropriate forces. Such materials may serve numerous purposes, including athletic footwear, heart stents, and aircraft wings.
“Origami has garnered considerable interest over the last decade due to its capability to deploy or transform structures,” stated James McInerney, principal author of the recent study, who conducted the research as a postdoctoral fellow at the University of Michigan. McInerney is now associated with the National Research Council at the Air Force Research Laboratory.
“Our group contemplated how various kinds of folds could be utilized to manage how a material reacts when subjected to diverse forces and pressures.”
McInerney and his associates have introduced an innovative approach to modeling folds, aiming to gain a deeper insight into how they can influence a material’s characteristics, which is a surprisingly intricate issue.
The underlying concept is similar to how a creased piece of cardboard behaves more predictably than an unmarked piece that may buckle in varied ways under pressure. By incorporating folds, the researchers can refine how materials respond to force. McInerney mentioned that the applications of such control are extensive.
“There are various scenarios, from the architecture of buildings, planes, and naval crafts to the packing and transportation of goods, where a balance often needs to be struck between enhancing load-bearing capacities and increasing overall weight,” McInerney said. “Our ultimate aim is to improve load-bearing designs by integrating origami-inspired creases—without adding additional weight.”

Recently published in Nature Communications, the study also encompasses Zeb Rocklin, McInerney’s doctoral mentor at the Georgia Institute of Technology; Xiaoming Mao, a professor of physics at the University of Michigan; Glaucio Paulino from Princeton University; and Diego Misseroni from the University of Trento.
“In a broader sense, this origami represents an instance of ‘metamaterials’—engineered substances that attain novel properties through structuring rather than merely chemical composition,” Mao expressed. “The geometry of folding—simple to realize in practice—grants a sheet of paper entirely new functionalities.”
Below the fold
Although planar materials, such as sheets of paper, are straightforward to envision, their behaviors under stress are intricate.
“If I pull on either side of a piece of paper, it remains solid—it doesn’t split,” explained Rocklin, associate professor of physics at Georgia Tech. “But it is also pliable. It can crumple and undulate depending on how I maneuver it. That presents a significantly different behavior compared to what we might observe in a typical solid, and it’s quite advantageous.”
Incorporating creases can “program” the materials to behave in a specific manner; however, figuring out how and when to make those folds poses a hurdle, even for contemporary physics.
“With these materials, predicting outcomes is often challenging—how the material will deform under stress, as they can take many different forms,” Rocklin asserted. “Traditional physics techniques struggle to address this type of issue, which is why we continue to develop new methods for characterizing structures in the 21st century.”
When it comes to origami-inspired materials, physicists start with a flat sheet that is methodically creased to create a designated three-dimensional form. Yet, this approach has its constraints. Previous models primarily concentrated on parallelogram-based folding, which uses shapes such as squares and rectangles, thus allowing for restricted types of deformation.
Consequently, Rocklin, McInerney, and their team explored trapezoids, which feature only one set of parallel sides. Utilizing these more variable shapes complicates the modeling of this type of creasing, yet enhances versatility.
“Through our models and empirical tests, we discovered that trapezoidal faces exhibit a wholly different classification of responses,” asserted McInerney. And these responses yield new functionalities, he added.
The designs possessed the capability to alter their shape in two unique manners: “breathing” by uniformly expanding and contracting, and “shearing” through twisting deformations.
Unexpectedly, the researchers also found that some behaviors seen in parallelogram-based origami transferred to their trapezoidal origami, suggesting some characteristics might be universal across different designs.
“While our research is theoretical, these revelations could present us with additional opportunities on how we might implement these structures and utilize them,” Rocklin remarked. “It’s a notably complex issue, but biology and nature are filled with ingenious solids—including our own bodies—that deform in particular, beneficial ways when necessary. That’s what we aspire to replicate with origami.”
This investigation was financed by the Office of Naval Research, the European Union, the Army Research Office, and the National Science Foundation.
Written by Selena Langner
“`